Interactive World Map of Research of Block Copolymers
Jocelyn Chin
August 2021
Summary
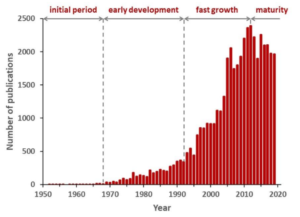
Research on block copolymers first began in 1952 and has greatly increased in recent years as shown by the increased number of publications in the past two decades.1 As research has evolved within this field, the United States, China, and South Korea have been taking a large portion of the research publications and collaborations.1 However, international collaboration in research is important to increase diversity of thought, educate scholars worldwide, aid other nations in scientific goals, and develop relevant technologies throughout the world. Thus, this internationally focused project contains a world map resource that highlights research groups and laboratories that have been pursuing block copolymer research and their relevant technologies in hopes to encourage greater international collaboration with a diverse set of scholars.
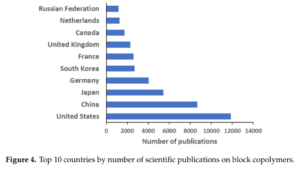
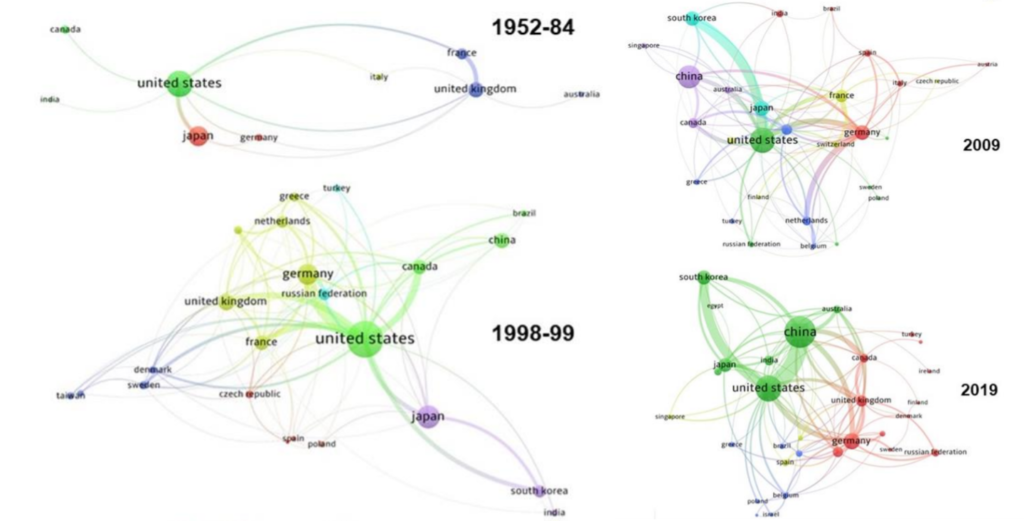
Brief background and Overview of history
A block copolymer (BCP) is a type of polymer made by joining types of polymer chains by a covalent bond.2,3 Because of their abilities to self-assemble into ordered nanostructures, they have various applications in nanotechnologies, soft lithography, thermoplastic elastomers (TPEs), and drug delivery.4 Thermoplastic elastomers, specifically, can be found in everyday household items such as adhesives and food packaging.5 Meanwhile, block copolymers also have applications in lithography through fabrication of microelectronic devices.6 These applications can be found worldwide, thus highlighting the importance of this research on an international level.
To utilize BCP in these applications, they must be self-assembled into ordered nanostructures. To control the self-assembly of BCP, thermal annealing and solvent vapor annealing are commonly used. Thermal annealing is done by raising the temperature of the thin film above its Tg and then cooling the film down to room temperature for further experiments.2 Solvent vapor annealing (SVA) is another common approach to organize and rearrange the polymer molecules. Compared to thermal annealing, SVA is a milder & non-destructive technique that is more convenient because it can be tuned precisely by altering the solvent characteristics and its vapor pressure. The samples do not have to be heated above Tg for long intervals and instead are subjected to solvent at room temperature for short periods of time. For over two decades, solvent vapor annealing has been shown in previous research to improve self-assembly of block copolymer films.7
The map below illustrates different groups who have pursued block copolymer research with various techniques. Since several variables can change the outcome of self-assembly, research throughout the past decades occurred concurrently and as a result of one another with various alterations in variables, polymer type, and solvent. Thus, this map is a brief, and non-extensive, overview of past advancements in this field.
Techniques used with discoveries in this area
To monitor changes in the self-assembly of block copolymers or morphology in general, there are several methods that researchers have used. These techniques are not unique to the field of polymer science but are popularly used to measure changes in block copolymers.
Spectroscopic Ellipsometry
Spectroscopic Ellipsometry (SE) is a fast (1s/measurement), accurate (+/- 1nm), and non-destructive technique that can measure optical properties and thickness of thin films of the order of a few nanometers to micron thick. This tool is useful in block copolymer work to measure the thickness changes of the films and change in optical properties, such as birefringence, to detect changes in morphology.8
Grazing Incidence Small Angle X-ray
Grazing Incidence Small Angle X-ray (GISAXS) is a photon scattering technique that can be used to find the size, shape, and alignment of nanoscale features of a flat sample substrate. It is used to show the lateral dimensions and arrangements of thin films.
Grazing Incidence Small Angle Neutron Scattering
Grazing Incidence Small Angle Neutron Scattering (GISANS) is like GISAXS but utilizes scattering of neutrons rather than photons. This technique is used to show the structure and morphology of surfaces and films. Lateral structures can be probed without damage and average statistical information over the surface can be found.
Atomic Force Microscopy
Atomic Force Microscopy (AFM) is a scanning probe microscopy that images the surface topography of a sample on the nanometer scale. Using a laser beam and deflection system, AFM can quantitatively and qualitatively measure the surface of polymers which can illustrate changes in morphology.
Transmission Electron Microscopy
Transmission Electron Microscopy (TEM) is an imaging technique that produces high-resolution images as a beam of electrons pass through the same to produce the image. The electrons that pass through the sample provide a resulting image that can show thickness/density, composition, and sometimes crystallinity.
Scanning Electron Microscopy
Scanning Electron Microscopy (SEM) is a technique that can determine the morphology, chemical composition, crystallization, and orientation of materials in a sample. It utilizes a beam of high-energy electrons that interact with the sample to get this information.
World map with Research of BCP
International collaboration is important for a variety of reasons as it helps conduct new avenues of research, increase diversity of thought, and educate scholars and researchers. Additionally, more widespread research aids other nations in scientific goals and helps to develop relevant technologies.
A solution to the disparity in worldwide research is to create an interactive tool that contains various laboratories working on block copolymers to encourage more diverse collaboration. Below is a prototype of a map containing theses laboratories. In the future, the hope is that this map is expanded to allow principal investigators and institution leaders to update their own pins and for similar maps to be created for other research disciplines.
Prototype of an International Map of Research on BCP
This map would be interactive where users could hover over a pin and see a pop-up of information that includes Research Group Name, Host Institution, Overview, Equipment, Contact, and Website. The below demo is not an exhaustive list of all publications or laboratories/equipment but rather a sample to show the international reach of this research.
Information on demo map listed in Table format.
Future research avenues
Collaborations between laboratories with various equipment can be helpful to gain a better understanding of block copolymers. Due to the interdependent variables associated with solvent vapor annealing for block copolymer self-assembly, there has recently been an increased interest in utilizing machine learning along with traditional techniques9. A local collaboration that the Fakhraai laboratory is pursuing is a partnership with Brookhaven National Laboratory to conduct in-situ ellipsometry and in-situ GISAXS with machine learning to find the optimal conditions for solvent vapor annealing of block copolymers to achieve long-range ordering. However, similar collaborations can be expanded to a worldwide level with partnerships with places like the African Lightsource. Thus, this worldwide map is a tool and gateway for future international collaborations.
Brief Overview of Research project that stimulated this project
Diblock copolymers (BCP) self-assemble into highly ordered nanostructures upon solvent vapor annealing (SVA) and this property of BCP has a wide range of applications. Recent research has shown that the kinetics of self-assembly can be monitored by measuring the change in birefringence in-situ, using spectroscopic ellipsometry. For the system, polystyrene-block-poly(ethylene oxide), PS-b-PEO, room temperature lies between the glass transition temperature (Tg) of each polymer block which results in the BCP existing in a partially glassy and partially rubbery state. We demonstrate that this semi-rubbery and semi-glassy system at room temperature disorders upon SVA, unlike other BCP systems and only self-assembles upon quenching. We are first establishing the conditions for SVA to find the optimal conditions for self-assembly of the BCP and then, we are working to understand the driving force and kinetics of the self-assembly process for this system under different temperatures.
Bio
Jocelyn Chin is a junior studying Chemical and Biomolecular Engineering at the University of Pennsylvania and double minoring in Sustainability and Environmnetal Management and Engineering Entrepreneurship. She has been involved with the Fakhraai research lab within the Chemistry department since her freshman year and has worked on projects including designing a temperature-controlled stage using SolidWorks, contributing to the Penn Glass Database, and most recently studying the self-assembly of block copolymers using in-situ ellipsometry.
References & Acknowledgements
References
(1) Lazzari, M.; Torneiro, M. A Global View on Block Copolymers. Polymers (Basel). 2020, 12 (4). https://doi.org/10.3390/POLYM12040869.
(2) Wang, C. Design of Solvent Vapor Annealing Tool and Study of Kinetics of Self-Assembly Block Copolymers for Nano-Lithography, Lund University, 2020.
(3) Reusch, W. Polymers 1. https://www2.chemistry.msu.edu/faculty/reusch/VirtTxtJml/polymers.htm.
(4) Feng, H.; Lu, X.; Wang, W.; Kang, N. G.; Mays, J. W. Block Copolymers: Synthesis, Self-Assembly, and Applications. Polymers (Basel). 2017, 9 (10). https://doi.org/10.3390/polym9100494.
(5) Wang, W. Novel Thermoplastic Elastomers Based on Benzofulvene : Synthesis and Mechanical Properties, 2015.
(6) Poelma, J. E.; Ono, K.; Miyajima, D.; Aida, T.; Satoh, K.; Hawker, C. J. Cyclic Block Copolymers for Controlling Feature Sizes in Block Copolymer Lithography. ACS Nano 2012, 6 (12), 10845–10854. https://doi.org/10.1021/nn304217y.
(7) Cheng, X.; Böker, A.; Tsarkova, L. Temperature-Controlled Solvent Vapor Annealing of Thin Block Copolymer Films. Polymers (Basel). 2019, 11 (8). https://doi.org/10.3390/polym11081312.
(8) Bilchak, C. R.; Govind, S.; Contreas, G.; Rasin, B.; Maguire, S. M.; Composto, R. J.; Fakhraai, Z. Kinetic Monitoring of Block Copolymer Self-Assembly Using In Situ Spectroscopic Ellipsometry. ACS Macro Lett. 2020, 9 (8), 1095–1101. https://doi.org/10.1021/acsmacrolett.0c00444.
(9) Ginige, G.; Song, Y.; Olsen, B. C.; Luber, E. J.; Yavuz, C. T.; Buriak, J. M. Solvent Vapor Annealing, Defect Analysis, and Optimization of Self-Assembly of Block Copolymers Using Machine Learning Approaches. ACS Appl. Mater. Interfaces 2021, 13 (24), 28639–28649. https://doi.org/10.1021/acsami.1c05056.
Acknowledgements
I would like to acknowledge the Fakhraai group for their encouragement, support, and wisdom throughout my research. I would especially like to thank my mentor Shivajee Govind, and my principal investigator, Dr. Zahra Fakhraai. Additionally, I would like to thank Kristin Field for her help with the REACT program this summer.
This work was supported by National Science Foundation Partnerships in International Research and Education funding (Award # 1545884).